Policy library
Search and browse our reports, policy and evidence
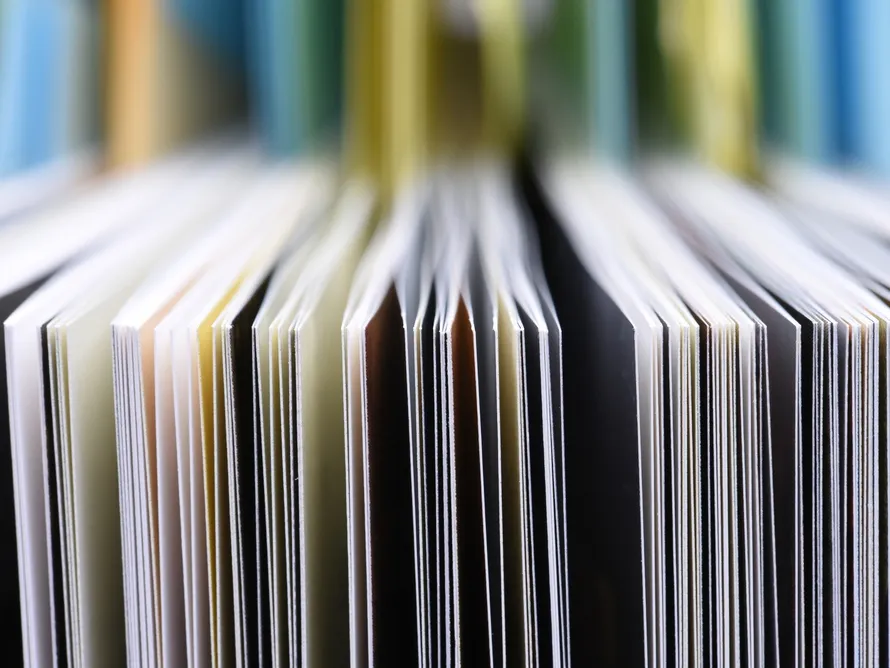
Our dedicated policy team, working with our members, wider community and expert partners, provide authoritative and robust information to shape current debates.
Whether maximising the effectiveness of research and education, or facilitating policymakers to use scientific information, our goal is to shape the development of policy relevant to the chemical sciences.
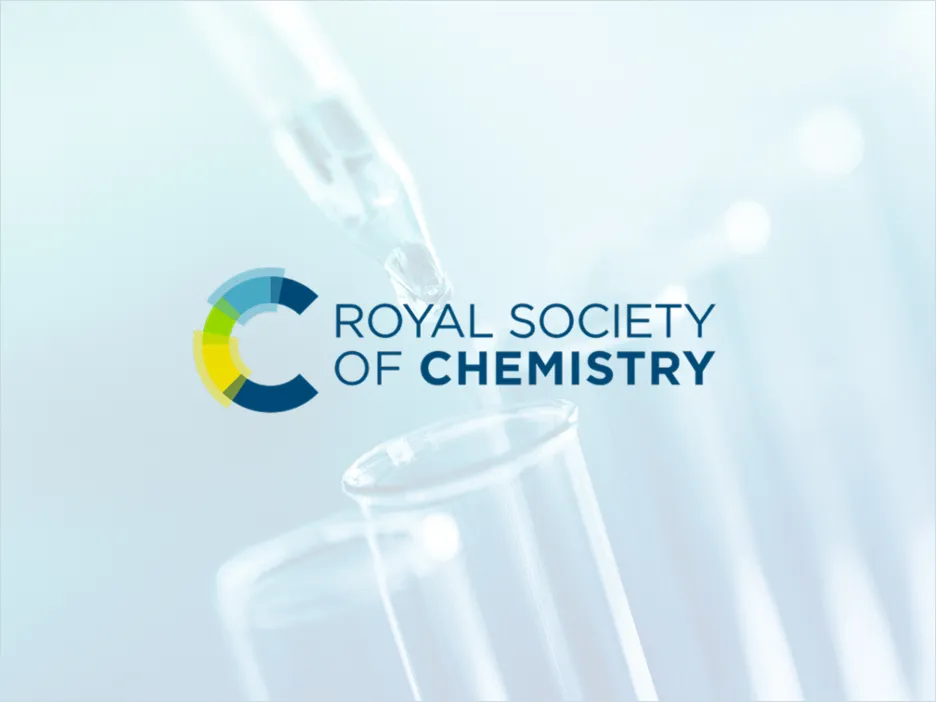
PFAS in UK drinking water
Download this policy position.
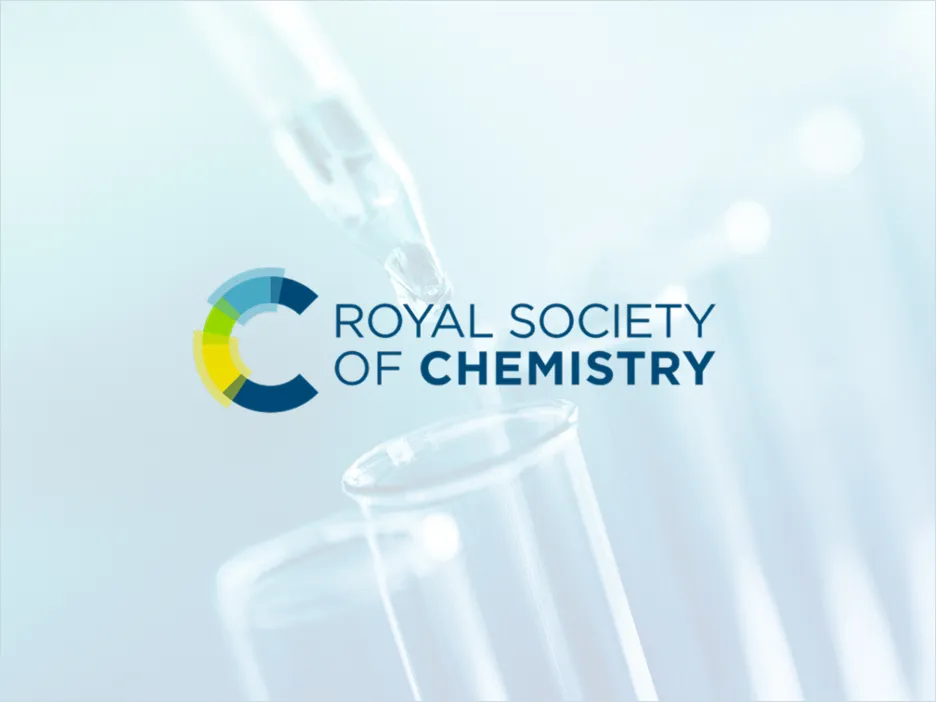
PFAS in UK waters
Download this evidence report.
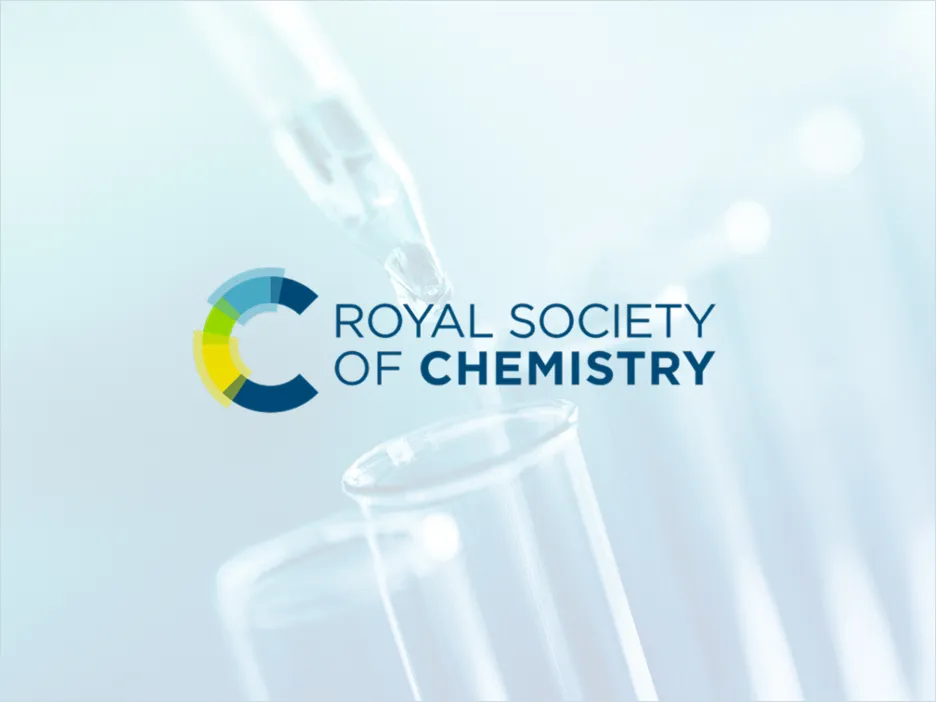
A landscape view of the global PLFs market
Download this report.
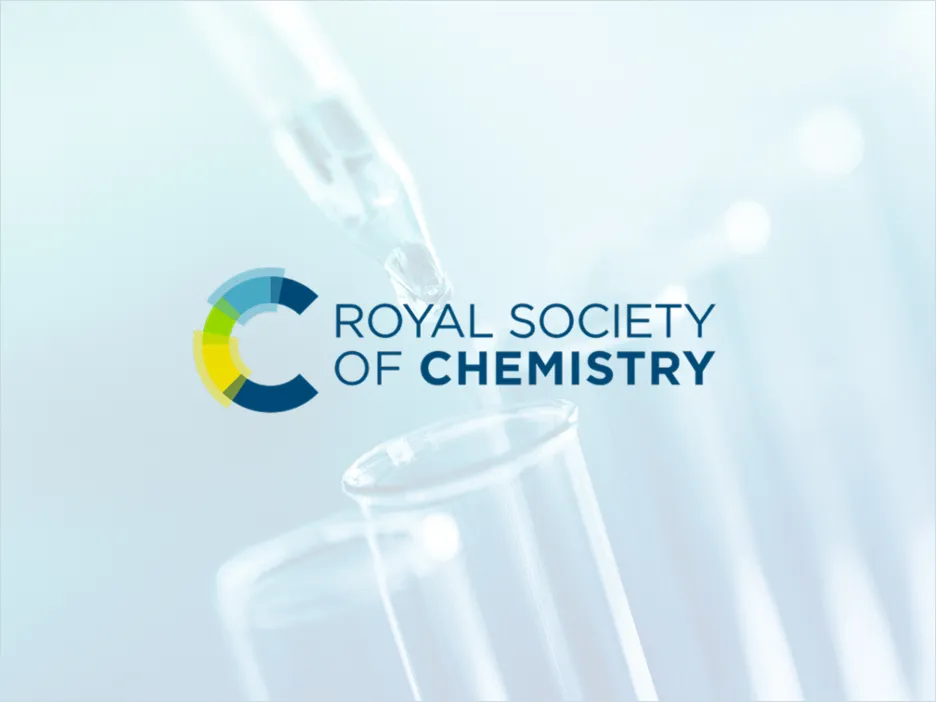
A circular economy for PLFs
Download this report
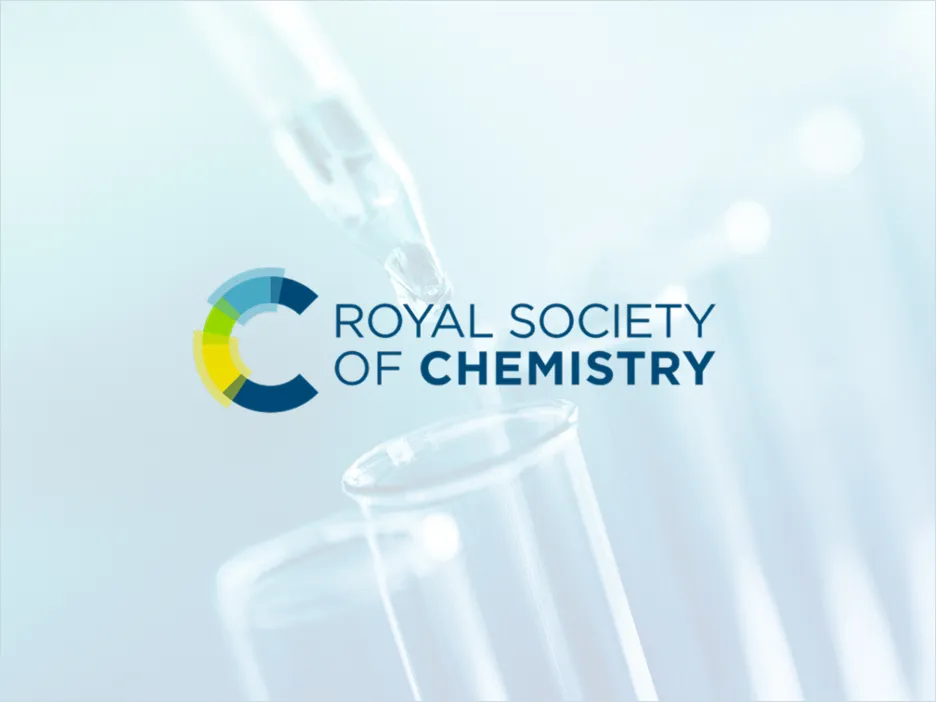
Environmental Audit committee inquiry on electronic waste
Download this evidence submission.
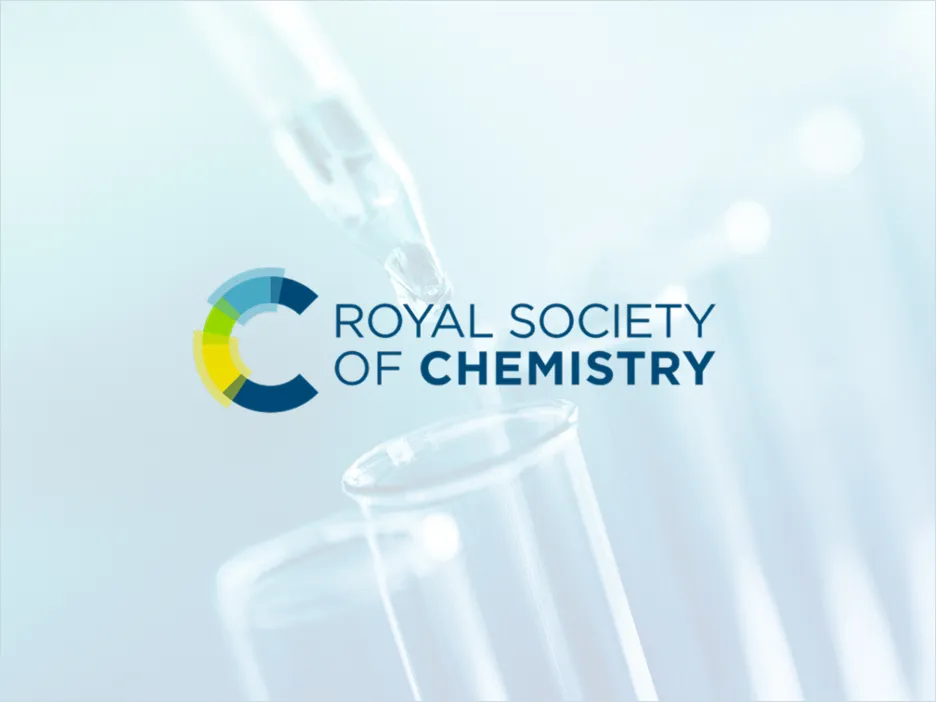
Reforming the producer responsibility system for WEEE 2023
Download this consultation response.
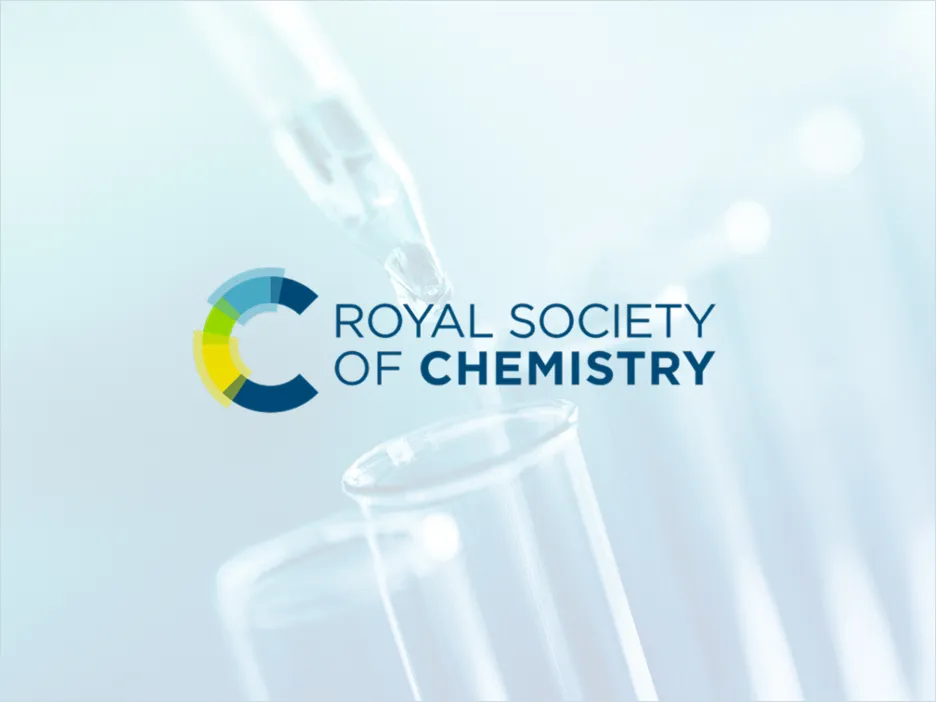
Not out of thin air
Download this report.
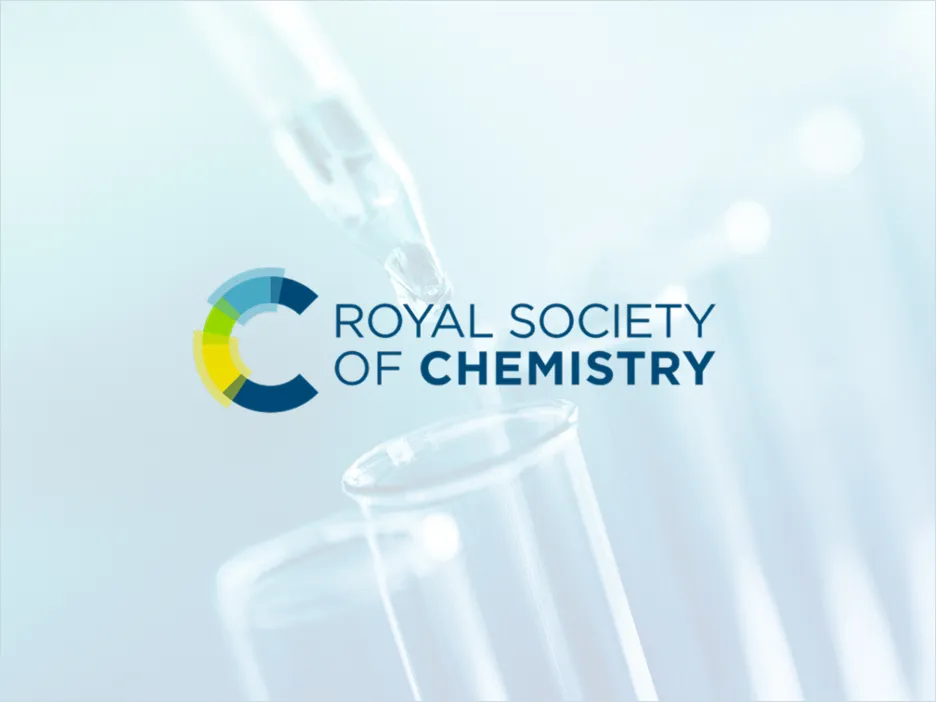
Chemistry-enabled sustainable composites
Download this report.
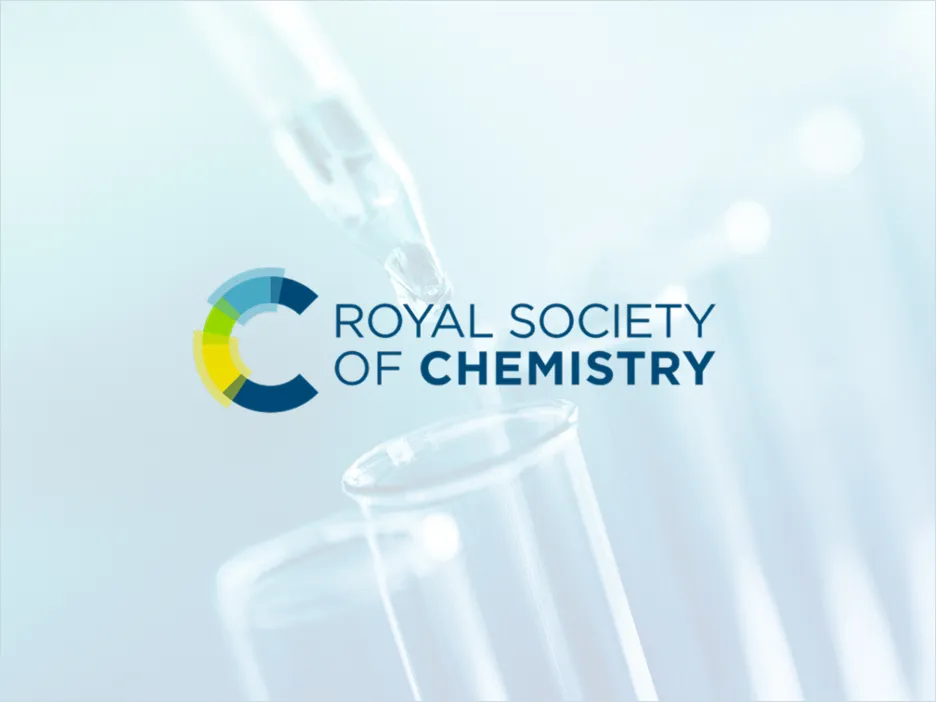
Sustainable laboratories
Download this report.
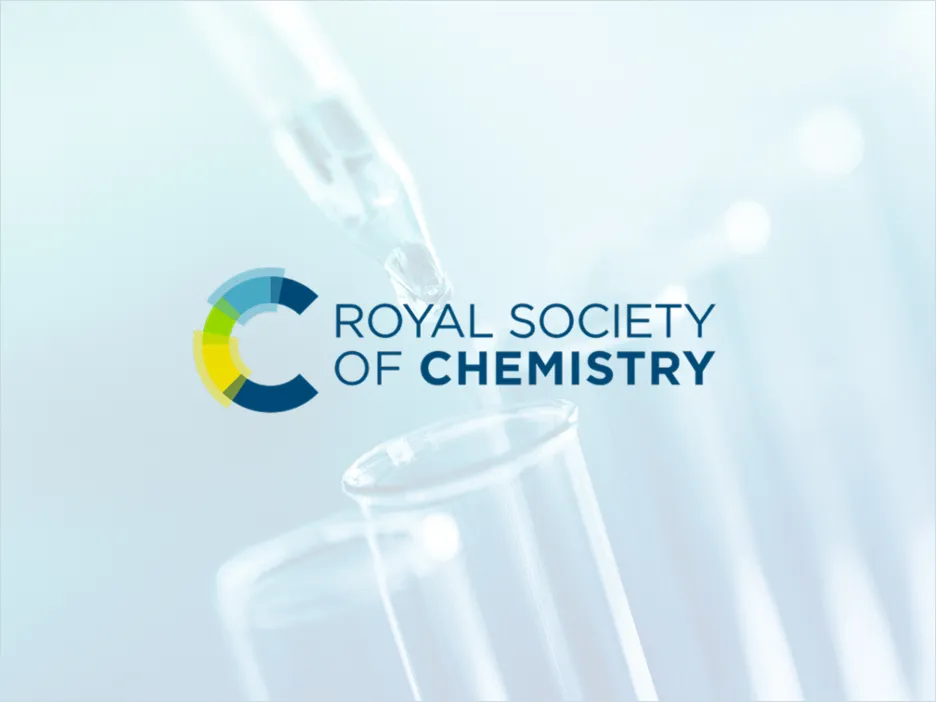
Plastic waste
Download this policy position.
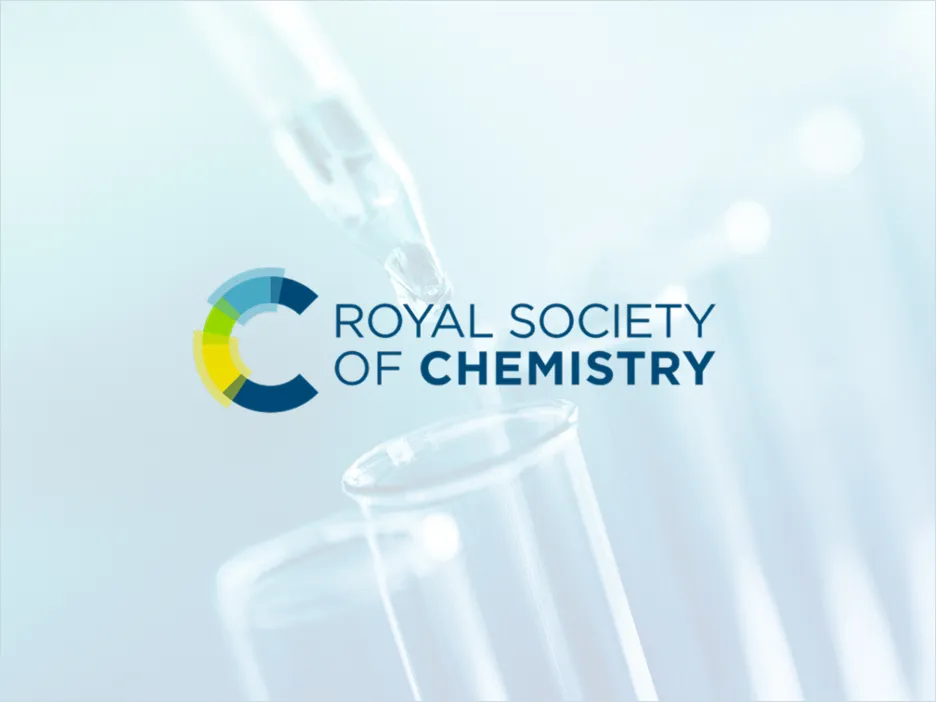
Green shoots: Part 1
Download this report.
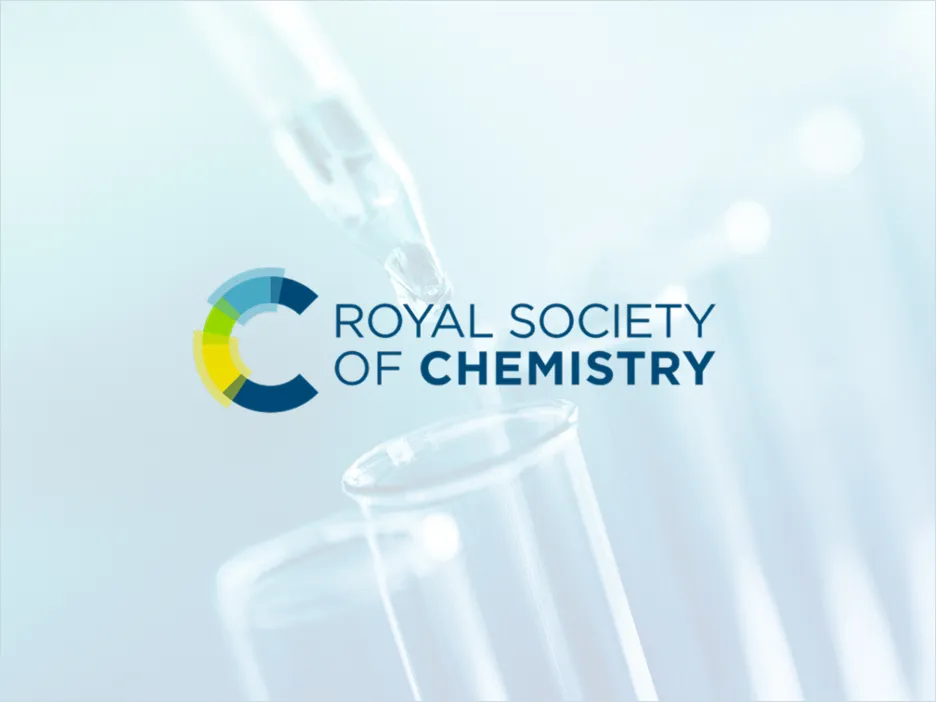
Green shoots: Part 1
Download this report.
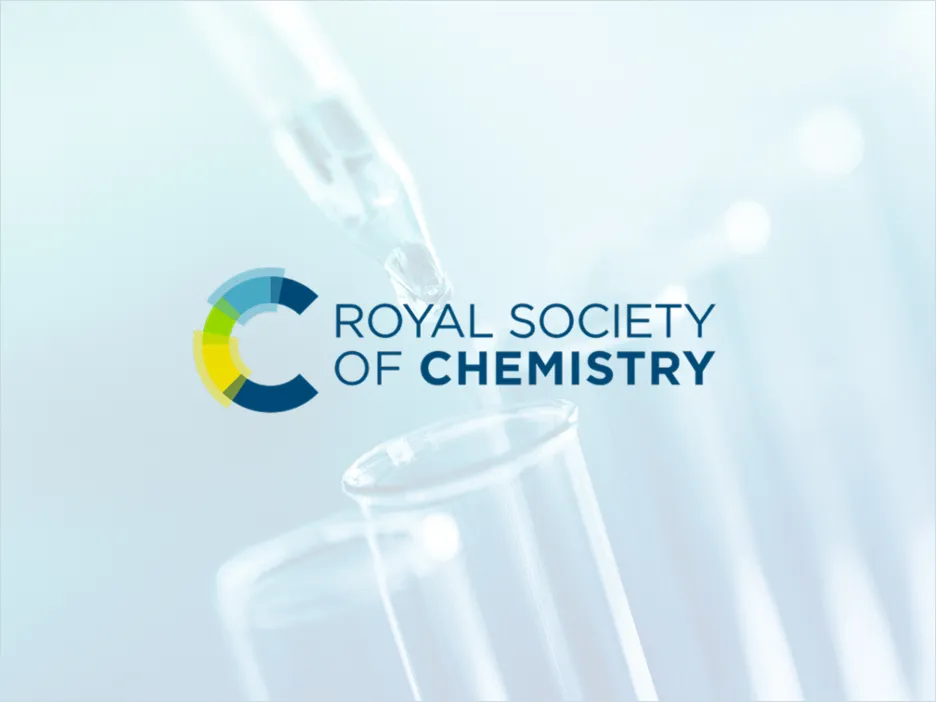
Drivers and scope for a UK chemicals framework
Download this report.
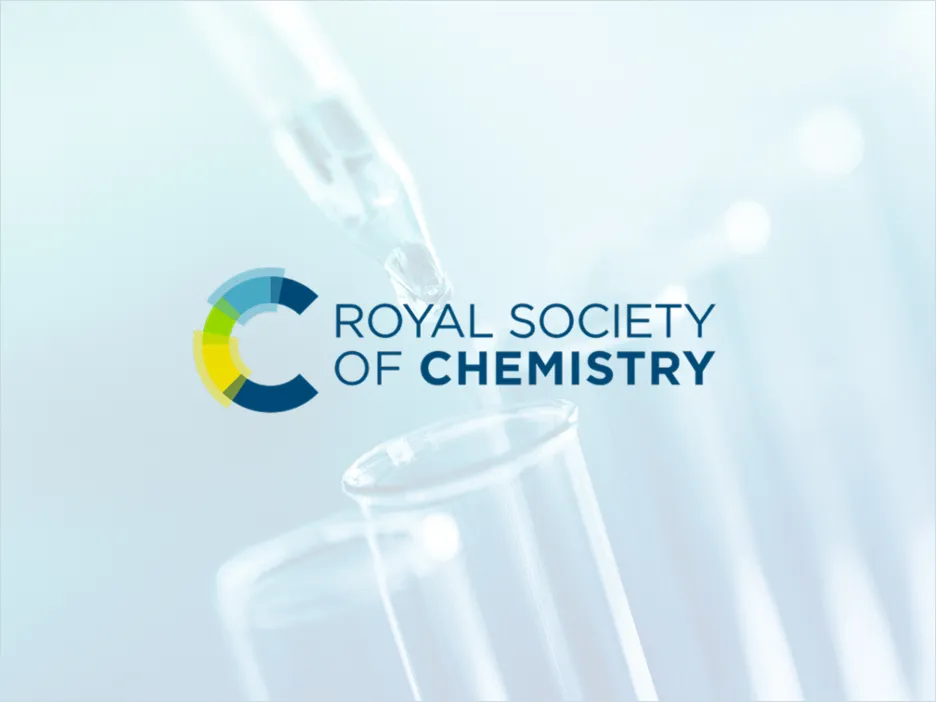
Tackling contaminants of emerging concern (CECs) in water
Download this policy position.
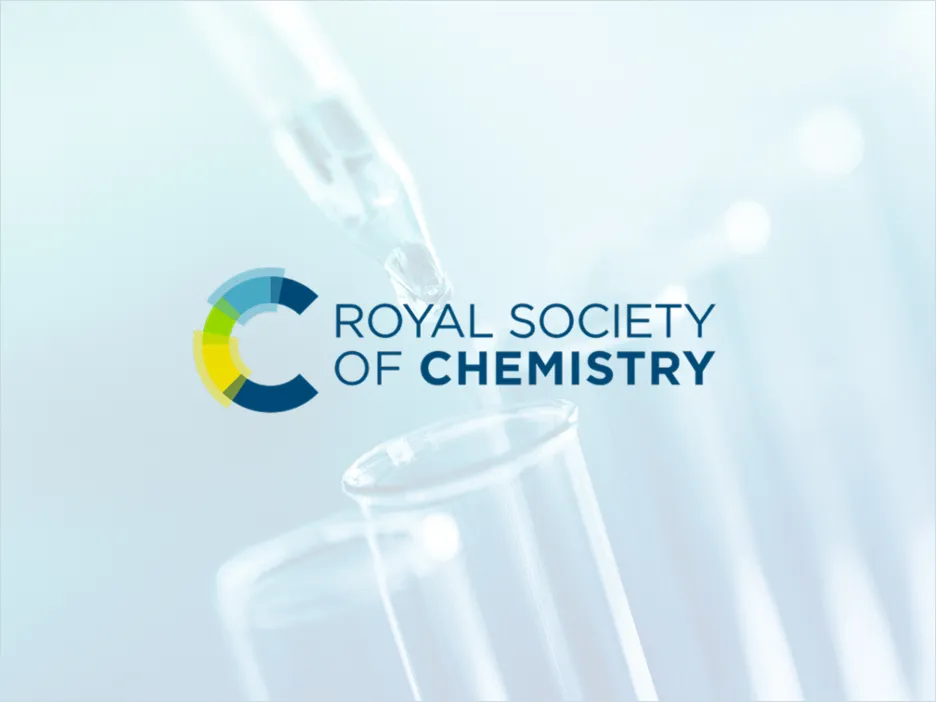
Stemming the tide of CECs in water
Download this explainer.